
Constituents in soils
L.A. Baker, B.C. Asleson, A.J. Erickson, and R.M. Hozalski
This section addresses the measurement of soil properties and soil constituents that are important to stormwater treatment practices. There may be several methods available for each analysis but only the most commonly used methods are discussed. Detailed description of the following analysis can be found in Methods of Soil Analysis Part 1 and Part 2 (Klute 1986, Black 1965).
Soil properties
Soils are an integral component for a variety of stormwater treatment practices and provide numerous functions for the treatment of stormwater runoff. Efficient treatment of stormwater runoff by soil processes requires properly functioning and stable soils. Therefore, measurement and understanding of soil properties are important for the overall assessment of stormwater treatment practices. Soil is composed of three phases: the soil matrix (solid phase), the soil solution (liquid phase), and the soil atmosphere (gaseous phase). The volume and mass relationships among these phases, along with some basic parameters, are useful to characterize the physical characteristics of the soil. The processes and the physical properties of the soil vary with surface location and soil depth and therefore soil analysis will typically require samples from several locations spatially at the soil surface and vertically within the soil profile.
Bulk density
Bulk density is the ratio of the mass of solids to the total soil volume. It can be used to estimate the degree of compaction and is needed to calculate soil moisture content and porosity. The bulk density of soil is influenced by soil structure due to its looseness or degree of compaction and by its swelling and shrinking characteristics (Hillel 1998). Soil compaction in stormwater treatment practices reduces infiltration by reducing the pore space available for water transmission. Soils in stormwater treatment practices can become compacted during construction. Post-construction soil compaction typically does not occur unless heavy machinery is used for maintenance/redevelopment of the practice or surrounding areas.
Bulk density is typically measured using the core method, which involves drying and weighing a soil sample of a known volume (Klute 1986). Bulk density can also be measured or estimated using digital or analog soil penetrometers, the sand cone test (A.S.T.M. D1556-90), nuclear density meters (A.S.T.M. D2292-91), etc.
Soil texture
Many of the physical and chemical properties of soil are affected by soil texture (Pepper et al. 1996). Soil texture is described by classifications which are determined by the particle size distribution of sand, silt, and clay within the soil. The particle size distribution of soil can be measured in the laboratory according to standards of the U.S. Department of Agriculture, the American Society for Testing and Materials, and the International Soil Science Society.
Pretreatment of the soil prior to particle size distribution analysis is required to improve the separation and dispersion of aggregates (Klute 1986). After pretreatment of the soil sample, the sand fractions are measured using mesh sieves of various-sized openings. The fraction of silts and clays can be determined using the pipet or hydrometer methods. The pipet method is a sedimentation analysis that relies on the relationship of settling velocity and particle diameter (Klute 1986). The hydrometer method is similar to the pipet method but makes use of a calibrated hydrometer for multiple measurements of the suspended sediment over time. The soil texture classification can then be determined from the USDA textural triangle given the percent sand, silt, and clay of a soil, as shown in figure 6.3. Alternatively, a field procedure for approximation soil texture (Thien 1979) is provided in figure 6.4.
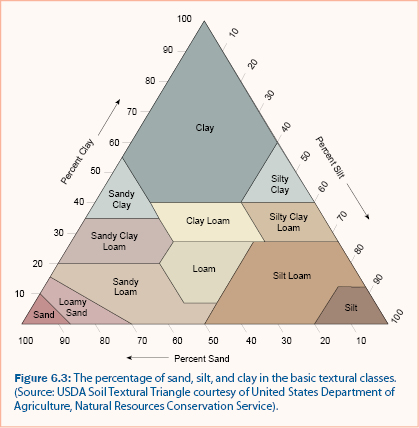
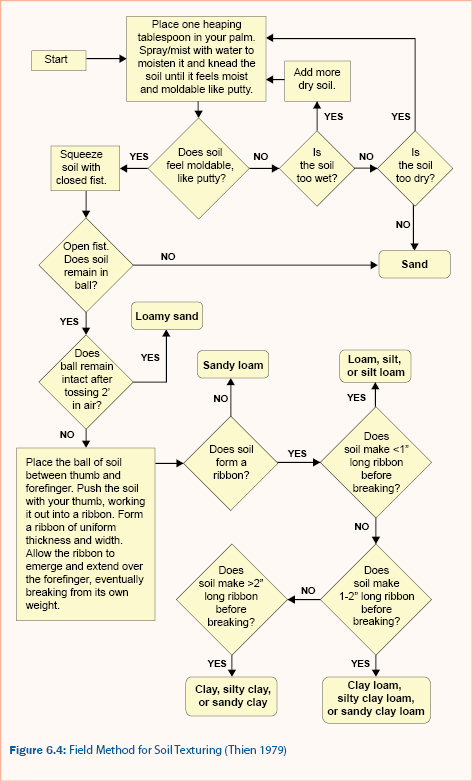
Soil texture analysis should also include identification of hydric soils. Hydric soils are formed when anaerobic conditions develop in the root zone due to prolonged saturation during the growing season (Richardson and Vepraskas 2001) and can be identified by the gray color of the soil and the presence of mottles. The gray color indicates a process of “gleying,” which includes the chemical reduction of iron or manganese. Mottles are small areas that differ in color (gray, red, yellow, brown, or black) from the soil matrix because of water saturation and chemical reduction. Reddish mottles, for example, are due to the accumulation of iron oxides in root channels or large pore spaces and black mottles indicate the accumulation of manganese oxides (Richardson and Vepraskas 2001). Hydric soils are evidence of prolonged water saturation, indicating that stormwater runoff is not infiltrating at sufficient rates. For more information on identifying hydric soils, refer to “Wetland Soils: Genesis, Hydrology, Landscapes, and Classification” (Richardson and Vepraskas 2001).
Porosity
The pore spaces in the soil matrix vary in amount, size, shape, tortuosity, and continuity and are an important physical property of the soil, especially with regard to the retention and transport of solutions, gases, and heat (Klute 1986). For stormwater treatment practices, the porosity is important because it is a measure of the soil’s capacity to infiltrate water. Porosity is the volume fraction of pores and ranges from 0.3 to 0.6 for most soils (Hillel 1998). Porosity (ƒ) can be calculated using equation 6.2 if the particle density (ρs) and the dry bulk density (ρb) are known. A typical mineral soil has a particle density equal to that of sand (2.65 g/cm3). Porosity can also be measured directly (Klute 1986) by saturating a known volume of soil, weighing it, and then drying it at 105 °C (221 °F). The difference in weights is the pore volume (the bulk density of water = 1.0 g/cm3) and therefore the porosity is equal to the pore volume divided by the original soil volume.
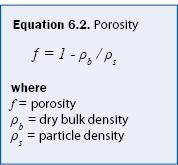
Penetrability
Compaction of soils can occur due to normal activities, can be induced by machinery, or both. Compacted soils exhibit small total porosity due to reduced volume and continuity of large pores which restricts aeration and impedes root penetration, infiltration, and drainage (Hillel 1998). Compaction can be estimated with penetrometers, which measure the ease with which an object can be driven into the soil. Penetration resistance measured by penetrometers is influenced by several soil factors including water content, bulk density, compressibility, soil strength, and soil structure (Klute 1986).
Water content
The amount of water in the soil influences numerous soil properties, governs the air content and gas exchange of the soil, affects plant growth, influences microbial activity, and dictates the chemical state of the soil (Hillel 1998). The measurement of water content may also be necessary for other assessment or analysis methods, such as determination of hydraulic conductivity of the soil with an infiltrometer or permeameter (see Infiltration). Prolonged saturation of the soil promotes the formation of hydric soils, while soil dryness can inhibit plant growth.
There are both direct and indirect methods for measuring water content. The traditional gravimetric technique involves weighing a fresh soil sample, drying the sample in an oven or microwave, and reweighing the sample to determine the amount of water removed. Indirect methods rely on the relationship between water content and certain physical and physical-chemical properties of the soil (Klute 1986) such as electrical resistance, capacitance, neutron scattering, gamma-ray absorption, and time-domain reflectrometry. There are several probes available for manually or automatically measuring water content in the field, with degrees of accuracy and calibration requirements that vary. Alternatively, visual (and tactile) assessment of the soil moisture can be made and the soil described as dry, moist, saturated, or inundated.
Hydraulic conductivity
Hydraulic conductivity of soil is a measure of its ability to transmit water and is used in Darcy’s Law to calculate flow or infiltration rates (Klute 1986). The terms “permeability” and “hydraulic conductivity” are sometimes used synonymously; however, permeability is an exclusive property of the soil matrix while hydraulic conductivity is also a function of the fluid properties (Hillel 1998). See Infiltration Practices for the discussion of the infiltration process and Infiltration for discussion and recommendations of devices used to measure hydraulic conductivity.
Cation exchange capacity
The cation exchange capacity of soil is a major sorption mechanism for pollutants and is due primarily to the negative charge associated with clay particles and organic particles. Positively charged ions (cations) such as heavy metals are attracted to the negatively charged sites on the clay particles, which influences the mobility of cations. As plants and microorganisms utilize cations in the soil solution, exchanges are made from the negatively charged particles (soil colloids) to the soil solution (Pepper et al. 1996) to balance the charge. The cation exchange capacity is the sum of the exchangeable cations in the soil and is usually expressed as milliequivalents of positive charge per 100 grams of soil (mEq (+) 100g-1). Common methods for measuring cation exchange capacity include saturating soil with a particular cation and then measuring the absorbed cations (Black 1965).
Soil pH
Soils with large concentrations of organic matter in areas with large rainfall tend to be acidic (pH < 5.5). The pH of the soil can influence the degree of ionization of compounds, especially heavy metals, which affects their solubility and may be critical to the transport of pollutants through the soil (Pepper et al. 1996). The measurement of soil pH is split into two methodic groups: colorimetric methods that utilize dyes or acid-base indicators and electrometric methods that utilize an electrode to measure the hydrogen ions (Black 1965).
Other soil properties
The analysis of other soil properties, such as water potential, evaporation rate, temperature, and air permeability, may also be desirable for assessment. For detailed standard procedures, see Methods of Soil Analysis (Klute 1986).
Soil constituents
Stormwater runoff carries various types of pollutants with it as it is conveyed. Measuring the type of constituents and their concentration in the soils of a stormwater treatment practice can be a useful assessment tool for understanding the soil’s capacity to retain those constituents. Retention (typically via sorption) is one of the major processes influencing the transport of pollutants in soil (Pepper et al. 1996). The retention and transformation of pollutants in the soil can prevent water quality degradation in lakes, streams, and rivers. To ensure that pollutants are retained and that the soil has not reached its capacity, analysis of the soils within the practice may be necessary. The mobility of pollutants and the physical properties influencing their transport vary spatially and therefore samples from several locations may be required to adequately characterize the pollutants in the soil. This section will discuss some of the key pollutants found in soils of stormwater treatment practices.
Organic matter
Plant residues incorporated into the soil surface are degraded by microorganisms into organic matter that is utilized by plants and microbes for metabolism and also incorporated into macromolecules (Pepper et al. 1996). Organic matter affects physical properties of soil such as bulk density, porosity and infiltration rate. The humic and nonhumic substances in organic matter contribute to the pH-dependent cation exchange capacity of the soil (see above) and the chelation of heavy metals. There are two approaches for determining organic matter content: loss-on-ignition and carbon content. Loss-on-ignition is a measure of organic matter volatilization and is determined by the difference between the dry weight of a combusted and non-combusted sample. Measuring organic carbon (OC) content is commonly done by measuring the production of CO2 during high-temperature combustion. Organic matter is then estimated using a ratio of carbon to organic matter (C:OM) which is often considered approximately 0.5.
Salinity (including chloride)
Soil salinity refers to the concentration of soluble salts within the soil. Salinity is important because it can harm plants by interfering with water uptake or through direct toxicity of ions associated with salinity (especially chloride). The accumulation of salts in soils also indirectly affects soil properties such as swelling, porosity, water retention, and hydraulic conductivity (Hillel 1998). A major source of salinity in stormwater treatment practices is road salt.
A common method for measuring salinity is by electrical conductivity which is typically expressed as millimho per centimeter (Black 1965). According to the U.S. Department of Agriculture Handbook 60 (Richards 1954), a saline soil has an electrical conductivity exceeding 4 mmho/cm at 25 °C (77 °F) (Hillel 1998).
Phosphorus
Phosphorus is retained in soils by adsorption and chemical precipitation. The accumulation of phosphorus in soils may need to be measured in stormwater treatment practices to assess whether the soils are saturated with phosphorus. The most commonly used phosphorus adsorption metrics are the Bray extraction method (for non-calcareous soils) and the Olsen method (for calcareous soils) (A.E.S. 1988). The relationship between extractable phosphorus in soil and phosphorus capacity is discussed in Impacts of urban stormwater.
Nitrogen
Total nitrogen is separated in several common forms: particulate N, nitrate (NO3-), nitrite (NO2-) and ammonium (NH4+). Plants typically use nitrogen as ammonium (NH4+) or nitrate (NO3-), which are generated directly from dissolving salts or indirectly through processes such as nitrogen fixation (conversion of atmospheric nitrogen to ammonia) or nitrification (oxidation of ammonia and ammonium to form nitrate) (Pepper et al. 1996). Nitrate is very soluble and has the potential to contaminate groundwater. Nitrate in runoff is also the primary contributor to hypoxia (dead) zones in coastal areas of the oceans. A biological process called denitrification converts nitrate into nitrogen gas (N2) and is discussed in Biological processes. There are several methods of nitrogen analysis, and the appropriate technique should be chosen depending on the form(s) of concern.
Metals
Metals commonly found in stormwater are lead, zinc, copper, and cadmium. There is the potential for the accumulation of metals in stormwater treatment practices, especially in the soils of infiltration practices. “Total” metal content is determined by rigorous hot-acid digestion of soil samples with one of several strong acids (often nitric), generally with a catalyst. “Extractable” metals are measured using extractions with weaker acids, usually at room temperature. A detailed discussion of metals analyses is found in Standard Methods (A.P.H.A. 1998b).
Microbial populations
Soil contains billions of living organisms that are essential to biochemical transformations and the overall health of the soil. These organisms can also capture and convert pollutants from stormwater runoff that may filter or infiltrate through the soil profile. The major groups of organisms found in soils include bacteria, actinomycetes, fungi, algae, viruses, and macro fauna (Pepper et al. 1996). The abundance of microorganisms in the soil, and therefore pollutant biodegradation, is dependent on oxygen and nutrient availability, organic matter content, pH, redox potential, temperature, and soil moisture texture (Pepper et al. 1996). Due to the variability in the type of microorganisms present in the soil and the special requirements for each species, it is challenging to measure the entire biological community in the soil. The most-probable-number (MPN) method is an estimate of the population density that avoids direct measurement of actual colonies (Black 1965). There are, however, additional techniques for measuring specific microorganisms (Black 1865).
Continue to Quality assurance program.